How does plant medicine adapt to meet modern health challenges? Simon Mills explores herbal interactions with the endothelium, gut wall, microbiome and neuroinflammatory processes.
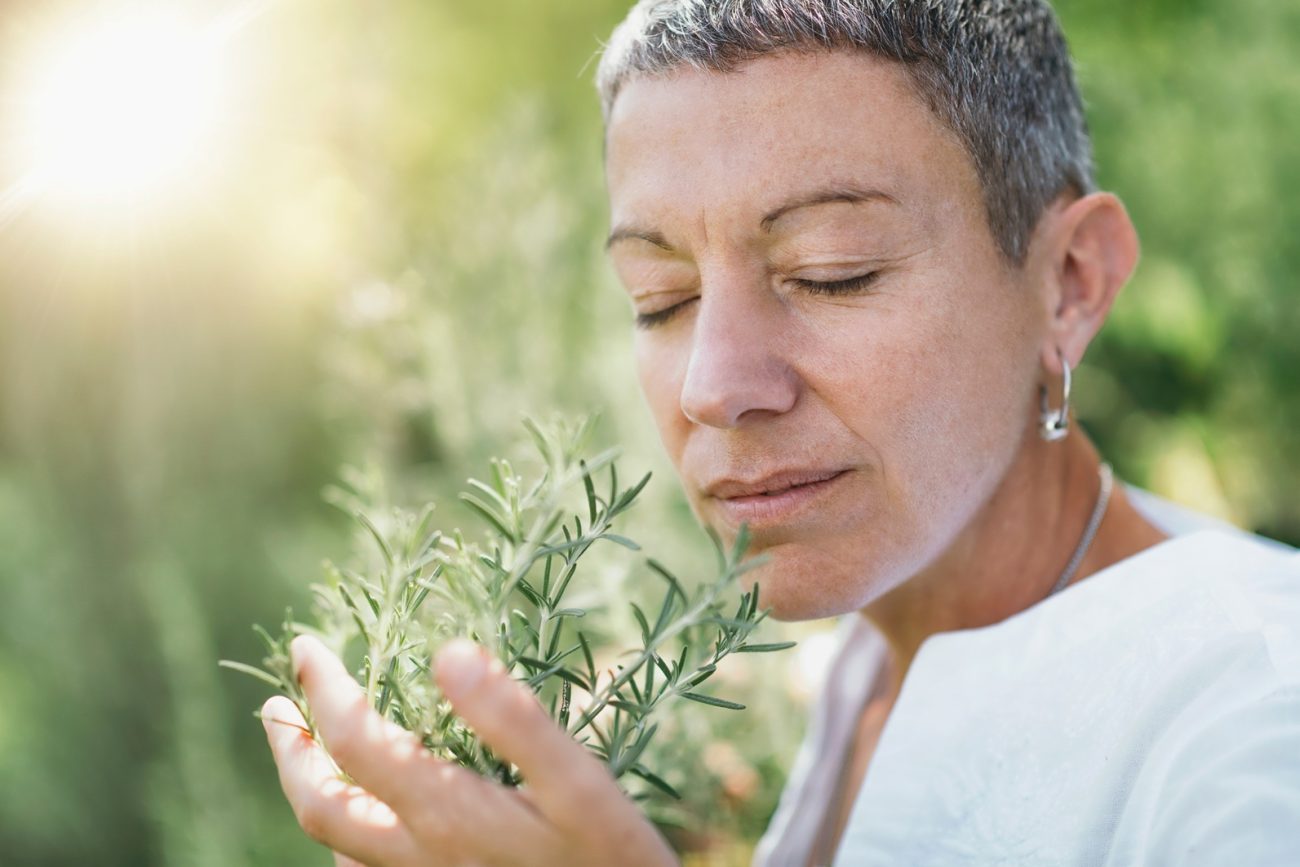
The use of plant medicines predates human history. They were there to remedy urgent health needs, pain, wounds, infections, fevers, infertility, and childbirth. Dosing was heroic because results were needed quickly. There was one simple outcome measure — survival! We can, therefore, be secure with those ‘human bioassay data’ as a basis for herbal efficacy. However, we can be less sure that these old remedies apply to our modern lives.
Our forebears would not have recognised hypertension or most cardiovascular problems, their exposure to dementia, diabetes and immune diseases was probably much more limited and arguably would have been rolled in to later life care or, as with mental disturbances, culturally managed in other ways. What confidence do we have, therefore, that the old remedies still work when all the health rules have changed? Is there any evidence that plants can be retooled to meet the new health care imperatives? How might we direct them to plug therapeutic gaps in modern society?
There are precedents for adapting traditional medicines to modern health challenges. In Europe, with both old traditions and relatively longer exposure to the post-industrial age, hawthorn moved from a fever management tool to a prescription for heart disease. Valerian and St John’s wort, both ancient convalescent tonics, were adapted to treat anxiety and depression. Garlic morphed from warding off the devil (and infectious diseases!) into a remedy to manage blood lipids. Twentieth century Germans transformed echinacea from a remedy used for the bites of snakes and venomous creatures, septicaemia and gangrene, to a gentle immune support; they also extracted a plant medicine never used in tradition at all — ginkgo leaf — and developed applications for it in dementia and circulatory disease.
So, let us look at whether we can further adapt the old plant medicines to fill some looming health priorities, largely ignored in classic texts:
- Diabetes and metabolic diseases
- Circulatory disease
- Dementia
- Mental health
- Immune and chronic inflammatory disease
Here are some cunning plant tricks that could hold the keys to real benefits. Although clinical evidence is still patchy, there are already strong indicators of promise. It is worth noting at the outset that most of these mechanisms can only be delivered meaningfully by plants. No other interventions can match them here.
How plant medicine impacts the endothelium
Blood vessel walls are lined with a single-cell layer, the endothelium. At the capillary level this is the wall. It is also the frontline in the instigation of inflammation. When healthy, it has a glassy surface and blood cells do not stick to it. If upset, its surface can get ‘sticky’ and white blood cells (leucocytes) start ‘tethering’ and otherwise engaging with it and become transformed in the process. In particular, they are activated to push their way between the endothelial cells and into the tissues behind (extravasation). Here, they play a key part in a range of inflammatory events to help dispose of the threat.
The following diagram shows some of the complexity of this process and some of the cytokines or chemical messengers that have been identified as critical (and are thus targets for anti-inflammatory drug development).
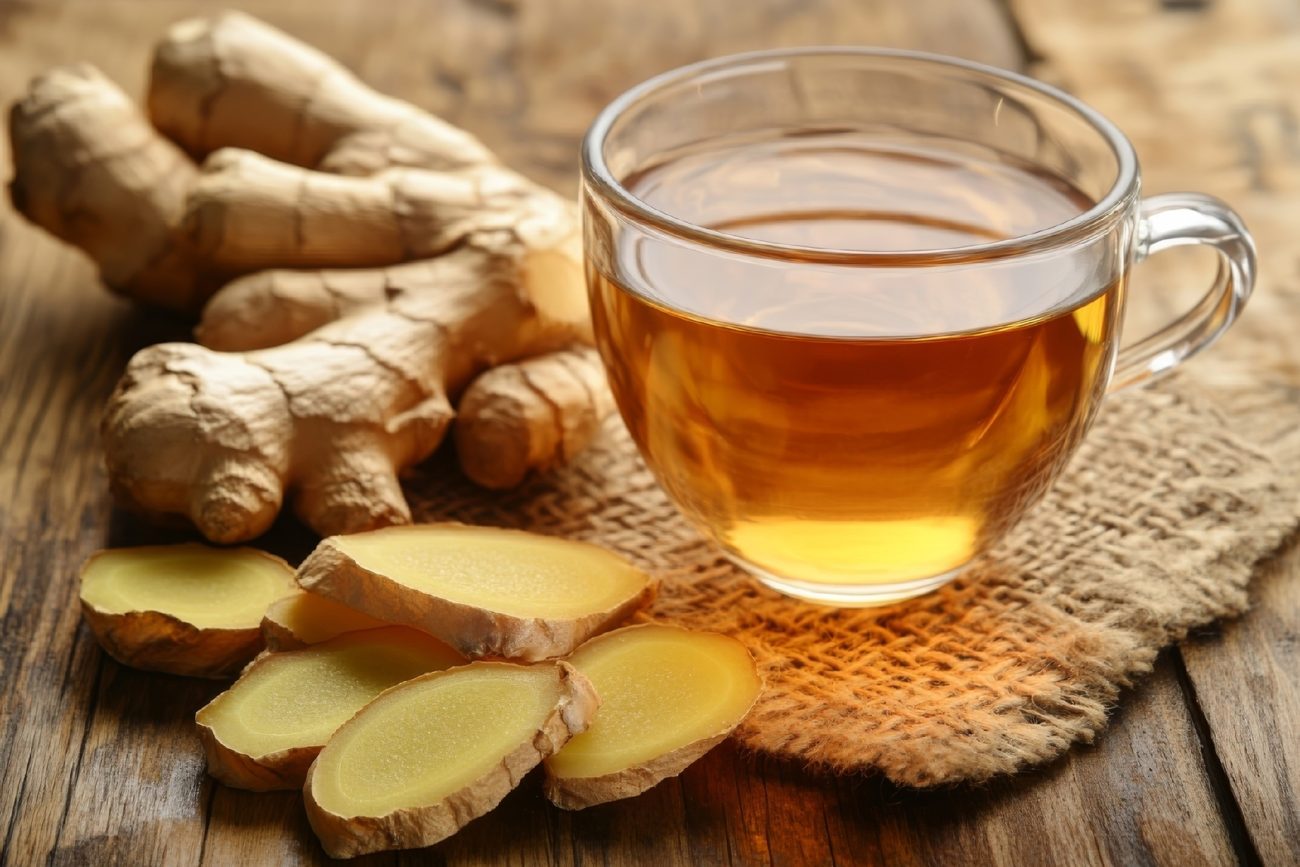
So, the endothelium is where inflammation often starts. How important is endothelial function or dysfunction to modern health?
Cardiovascular problems like heart disease, stroke, thrombosis, are all linked to atheroma formation (atherosclerosis). This has traditionally been associated mainly with hypertension, cholesterol and lipid disturbances, clotting disorders and blood vessel wall damage. It is now increasingly understood, however, that atheroma formation is at least as likely to be inflammatory in origin, with endothelial dysfunction at the core (1,2).
At least as relevant to modern ills, it is now understood that insulin resistance, the precursor to metabolic syndrome and diabetes, is essentially an inflammatory event at the endothelium. This contributes significantly to atheroma formation, affects blood pressure regulation, and other circulatory problems associated with diabetes and metabolic syndrome (3). Cutting a long and fascinating story short endothelial dysfunction is associated with a large proportion of modern health ills.
Even serious complications of Covid 19 infections such as micro-coagulations, myocarditis, and kidney disease, were early on associated with patients who had pre-existing cardiovascular disease, diabetes or obesity, and then not surprisingly linked to endothelial dysfunction (4).
An obvious conclusion is that insulin resistance, metabolic syndrome and their consequences mainly follow unhealthy modern diets, with excess refined carbohydrate consumption and processed foods. However, it does look as though plants have powerful counteractive activity. Spices have been studied for their potential effects on endothelial function, particularly in the context of meals high in fat and carbohydrates. A systematic review of clinical data is promising (5), and one study showed protection of moderate doses of culinary spices against a poor diet (6). Culinary levels of both Mediterranean herbs and Asian spices improved endothelial insulin sensitivity in overweight subjects within 24 hours (7), and similar quick responses on vascular function were seen after taking only one meal with curry spices (8). The incidence of retinopathy, one of the vascular complications of diabetes, has been significantly lower in Asian than Western countries (9). Some of the postulated protective effects of cinnamon include reducing insulin sensitivity at the endothelium (10). Among other spices, ginger intake significantly reduced markers of extravasation and affected systolic blood pressure in patients with type 2 diabetes mellitus (11).
All plants contain polyphenols and even higher levels are found in many remedial herbs and most spices. There is accumulating evidence that polyphenols can protect endothelial function (12,13), so reducing atheroma (14) counteracting metabolic syndrome (15,16) and reducing hypertension (17). A part of this impact is associated with higher local levels of vasodilatory nitric oxide production, as well as indirect effects of their impact on the gut microbiome (18).
The really important point about these data is that they relate to the use of medicinal plants in the diet. Herbal prescriptions can be appropriate for short-term benefits (as we have seen) but sustained protection against cardiovascular disease, metabolic diseases like diabetes and other chronic modern illnesses (see also later) relies on lifestyle factors, in which increased plant intake can clearly play its part.
How medicinal plants affect the gut wall receptors (‘acupharmacology’)
Herbal prescriptions utilise many plant constituents that have powerful effects on gut function primarily because they impact cells on the gut surface — and so many are poorly assimilable anyway. To appreciate the leverage this can bring we remind ourselves that most body functions are programmed to respond in coordinated ways to various inputs or stimuli — they do not perform haphazardly. Unlike single chemical medicines that are targeted at cell receptors, we can argue that plant remedies are blunter and less likely to reach the target, but well suited to ‘nudge’ these programmed responses. We can suggest that herbalists apply ‘reflex pharmacology’, at least as much as receptor pharmacology. Initiating systemic reflex responses across the body from the gut wall means there really is no need to go in and reach them. We can just poke in the right place and stand back!
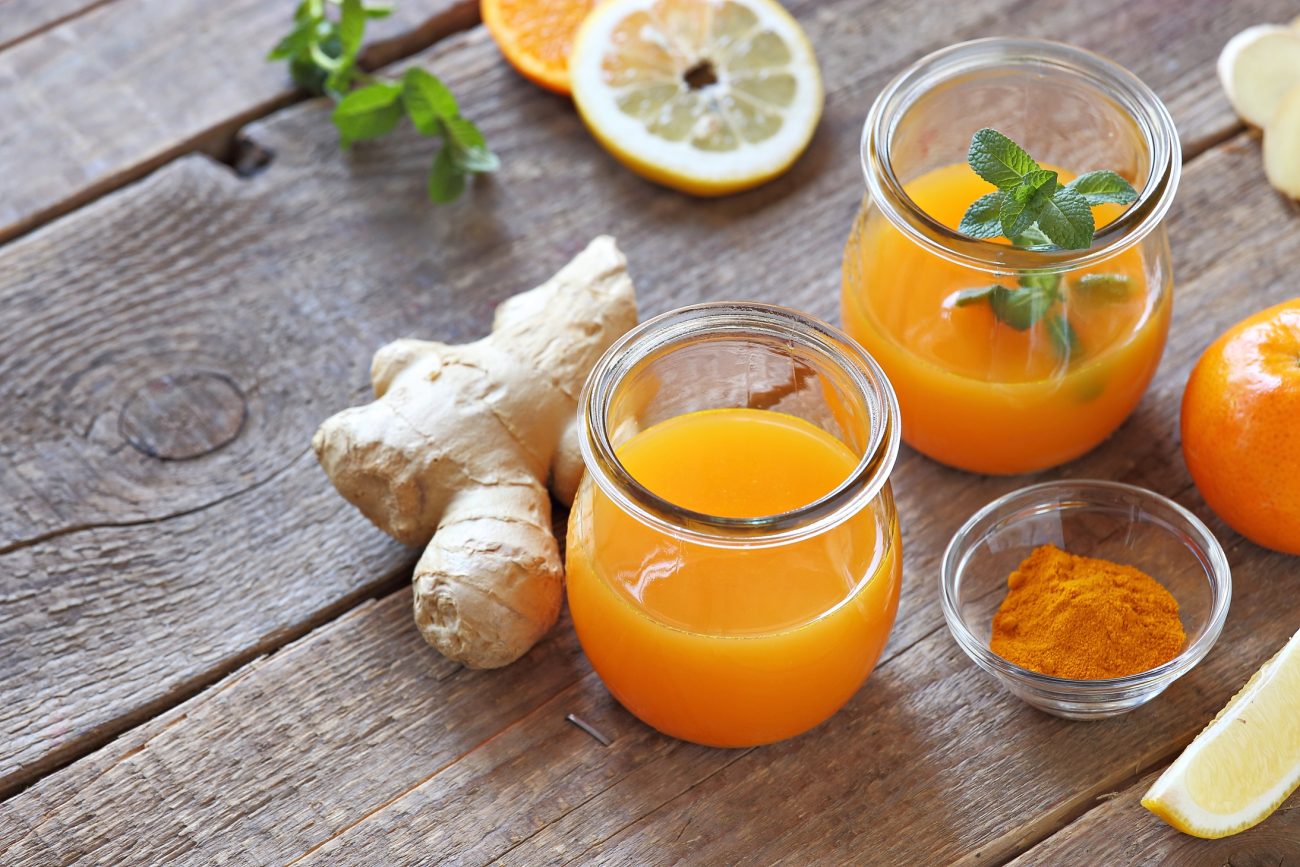
There is of course distinct value in working on the digestive tract, owing to the gastrointestinal system comprising,
- Most of the immune system
- A major site of hormone production
- The primary organ of elimination
- The home of the microbiome
- The source of nourishment and replenishment
- The basis of the gut-brain axis with many shared neurohormones
We summarise many of the mechanisms by which pant constituents initiate reflex responses in the ‘Acupharmacology’ section in our article on Digestive Health. An extensive range of references for this massive subject can be found in chapter 2 of Principles and Practice of Phytotherapy 2nd Edition.
The example of inflammation is particularly relevant to plugging modern therapeutic gaps. As we saw, so many pressing health issues of the day are in effect variations on chronic inflammation.
A wonderful example of this plant medicine action is the reputation of turmeric in potentially managing a wide range of inflammatory conditions around the body, in spite of the low bioavailability of its prime constituent curcumin (though see below). There is a growing body of evidence that shows how important curcumin is in reducing inflammatory pressures arising from the gut — in many cases the most significant origin of such trouble. One quote from a review paper says it well:
“Curcumin does not need to be absorbed to bring about its effects since it has profound effects on the intestinal wall and can effectively reduce inflammation by this mechanism” — Dr Shobha Ghosh Professor of Medicine and Physiology, Virginia Commonwealth University School of Medicine (19)
Plants and the microbiome can together generate potent postbiotics
Central to all gut considerations is the microbiome (and mycobiome — we need also to acknowledge the key role played by of our commensal fungi). The more we understand of the complexity of our resident flora the more we realise they are involved in almost all human physiologies.
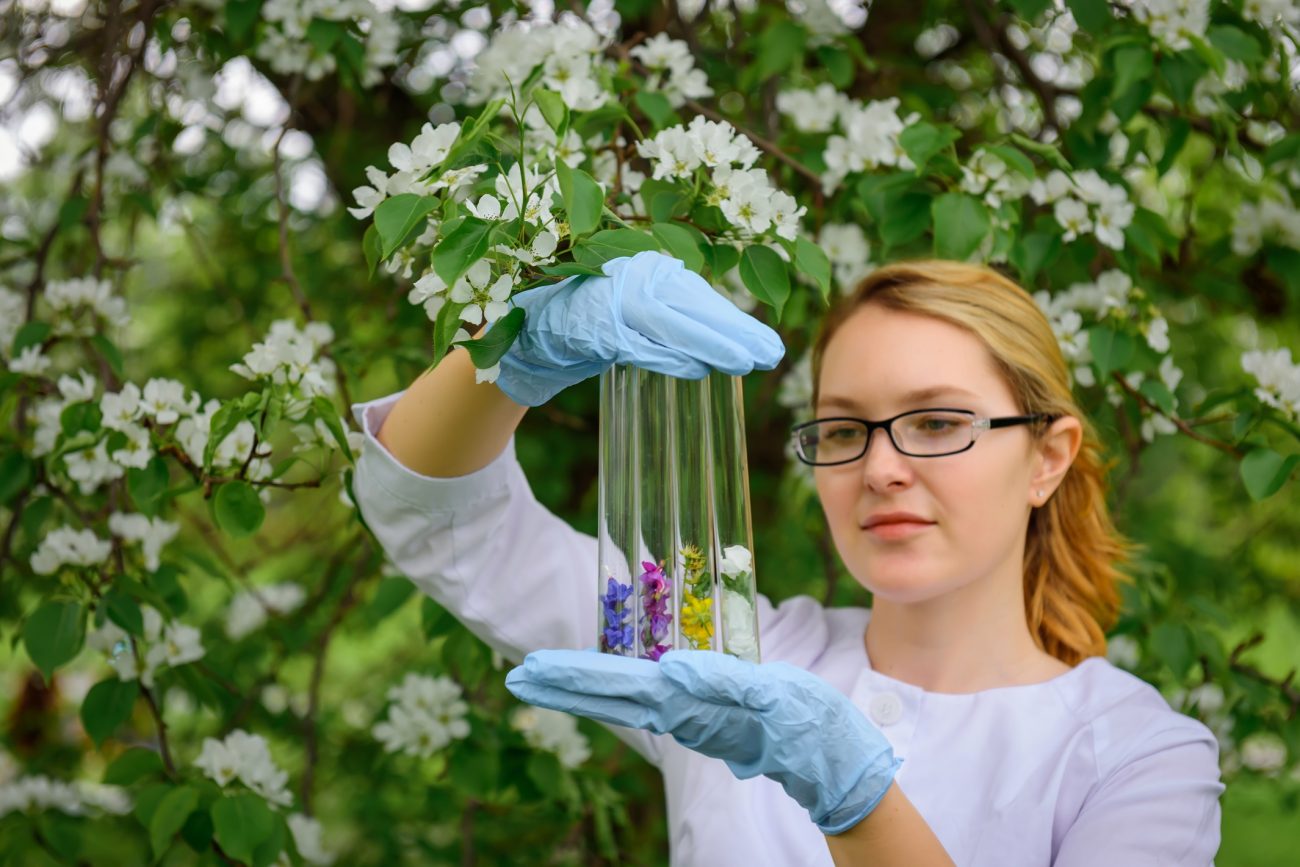
Many plant medicine constituents influence the gut populations as prebiotics. More, however, are processed by microbes into new active agents. The term ‘postbiotic’ has been developed to describe the metabolic products of industrial fermentation of probiotics, primarily intended for use as nutritional supplements (20). With literal interpretations to include all relevant microbial metabolites we can argue that the products of microbial metabolism of plant constituents also earn the description ‘postbiotic’.
Numerous studies implicate the gut microbiota in metabolising poorly absorbed, polyphenolic compounds from plants (21), including soy isoflavones, lignans from flaxseed and sesame seeds, flavonoids like the catechins and gallate esters found in tea, and ellagic acid from nuts and berries (22,23). These plant medicine molecules are processed using a range of transformations, including ring cleavage, demethylation, and dehydroxylation, which generally produce metabolites with higher oral bioavailability, increased bioactivity, and a correlation with lowered disease risk (24,25,26).
The classic example is how polyphenols are metabolised from relatively unassimilable large molecules to much more active and accessible simple phenols. To remind ourselves simple phenols are inherently bioactive (and include acetylsalicylic acid aka aspirin).
Curcumin also undergoes significant biotransformation by the gut microbiota, leading to the production of various metabolites (27), such as tetrahydrocurcumin (28,29). Human intestinal bacteria, such as Blautia spp. can demethylate curcuminoids, producing demethylcurcumin and bisdemethylcurcumin, with their own potential health benefits (30).
We have looked at the interaction between plants and the microbiome in our article Plants and the gut microbiome: Prebiotics and postbiotics
Again, the prospects, although still unravelling, hold promise for the natural management of chronic inflammatory diseases, and can explain some of the benefits being identified for polyphenols and other plant constituents in these conditions. Of course, this is a mechanism that only plants use.
Nrf2/ARE pathway activation
Although there is now justifiable pushback against the widespread use of the term ‘antioxidant’ in relation to natural remedies and supplements (firstly, all plants show antioxidant activity in vitro and secondly, few of these activities are translated into observable clinical benefits, mainly because thirdly, the action needs to be inside the cell rather than in the circulation), there is no doubt that intracellular antioxidant activity is vital for protection against oxidative pressures.
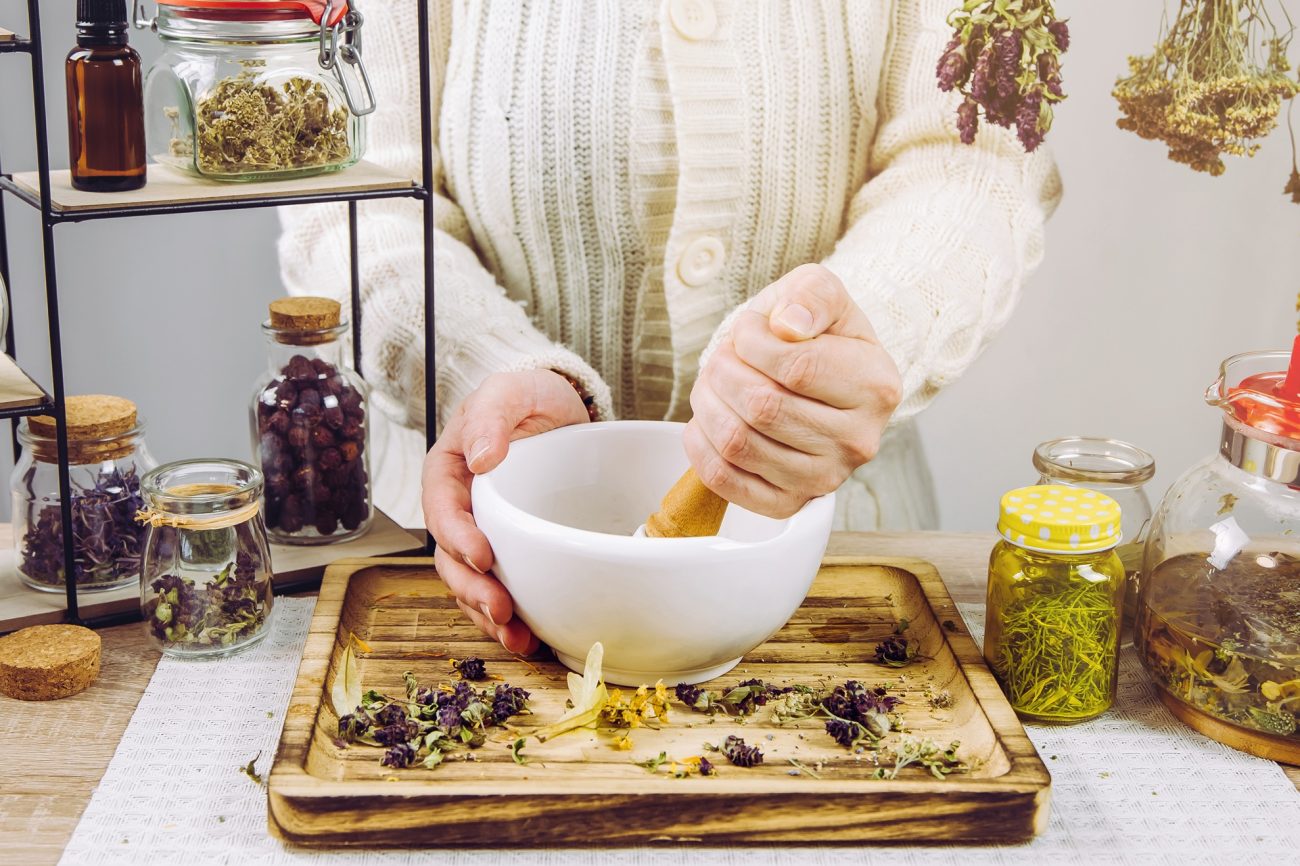
In the human body, reactive oxygen species (ROS) are produced as a normal product of cellular metabolism. Many ROS have unpaired electrons known as free radicals. In part, their role is useful, priming immune defences, for example, but by pairing with the polar elements of intracellular molecules, such as DNA and proteins, they can damage cell functions. Cells, therefore, need to generate their own antioxidative defences. These are mostly powerful enzymes such as catalase, superoxide dismutase (SOD), thioredoxin, or metabolites like glutathione. The value of enzymes is that they are produced on site and can catalyse millions more reactions than any nutrient or supplement.
Nuclear factor erythroid 2-related factor 2 (Nrf2) is such a defence. It is a ‘transcription factor’, a cellular chemical that induces the DNA to manufacture specific proteins. Chemical stress on the cell releases and activates Nrf2, it ‘translocates’ to the nucleus, and there binds to the antioxidant response element (ARE) of the DNA. This induces the synthesis of a range of important antioxidant, detoxifying, protective and anti-inflammatory enzymes: Nrf2/ARE pathway is therefore a general cell protection, extending beyond reducing oxidative stress.
One key area identified for the action of Nrf2/ARE is reducing ferroptosis. This is a recently discovered type of programmed cell death distinct from others, such as apoptosis, necroptosis, and autophagy. It is characterised by the accumulation of intracellular iron, overproduction of ROS, depletion of glutathione, and extensive lipid peroxidation in the cell membrane. Ferroptosis is linked with many problems such as neurodegenerative diseases, ischemia, cancer, and chronic kidney disease. Polyphenols, plant secondary metabolites known for many bioactivities, are being extensively researched in the context of their influence on ferroptosis (31,32).
Mobilisation of the Nrf2 pathway and reduction in ferroptosis has been particularly associated with plant medicine (33,34,25), especially polyphenols (36,37). Notable plants and constituents so far associated with Nrf2 activation are glucosinolates and sulforaphane from brassicas (38,39), coffee (40,41), green tea (42), turmeric (43), rosemary (44), garlic (45), ginkgo (46) and resveratrol from grape seed (47) among many others.
Another intriguing plant medicine story is the close link between Nrf2 activity and the bile-modulated FXR receptor. Connections are establishing with plant constituents, especially in connection with benefits on liver inflammation, importantly not requiring their absorption across the gut wall (48). Plant constituents are known to interact with FXR receptors in the gut wall (49), including polyphenols (50).
It is obvious from these new and exciting fronts in plant research that here are some powerful mechanisms for their use as foods and medicines for a wide range of modern inflammatory diseases.
Particular excitement has been generated in relationship to the benefit of plant-induced Nrf2 protection in reducing inflammation in the brain (51,52,53). There are other mechanisms identified, and the benefits for neuroinflammation deserves its own section.
How plant medicines dampen neuroinflammation
Inflammation in the central nervous system, across the blood-brain-barrier (BBB), is increasingly understood as a common factor in many intractable conditions:
- Chronic fatigue
- Fibromyalgia
- Depression
- Psychoses
- Dementia
This inflammatory breach of the BBB can be provoked by any source of inflammation in the body:
- Viral and ‘stealth’ infections
- Dysbiosis
- Ageing
- Autoimmunity
- Chronic cardiovascular disease
- Insulin resistance
As we have seen many of these are themselves amenable to plants and plant medicine.
We have posted substantial articles on the role of plants in neuroinflammation and on the blood-brain barrier in Herbal Reality. These will make clear that the potential for plant remedies and foods on this huge threat in modern society is among the most promising of all!
References
- Henein MY, Vancheri S, Longo G, Vancheri F. The Role of Inflammation in Cardiovascular Disease. Int J Mol Sci. 2022;23(21):12906. https://doi.org/10.3390/ijms232112906
- Theofilis P, Sagris M, Oikonomou E, et al. Inflammatory Mechanisms Contributing to Endothelial Dysfunction. Biomedicines. 2021;9(7):781. https://doi.org/10.3390/biomedicines9070781
- Barrett EJ, Liu Z. The endothelial cell: an “early responder” in the development of insulin resistance. Rev Endocr Metab Disord. 2013;14(1):21-27. https://doi.org/10.1007/s11154-012-9232-6
- Birnhuber A, Fließer E, Gorkiewicz G, et al. Between inflammation and thrombosis: endothelial cells in COVID-19. Eur Respir J. 2021;58(3):2100377. https://doi.org/10.1183/13993003.00377-2021
- Gupta K, Testa H, Greenwood T, et al. The effect of herbs and spices on risk factors for cardiometabolic diseases: a review of human clinical trials. Nutr Rev. 2022;80(3):400-427. https://doi.org/10.1093/nutrit/nuab037
- Petersen KS, Rogers CJ, West SG, et al. The effect of culinary doses of spices in a high-saturated fat, high-carbohydrate meal on postprandial lipemia and endothelial function: a randomized, controlled, crossover pilot trial. Food Funct. 2020;11(4):3191-3200. https://doi.org/10.1039/c9fo02438g
- Huang Y, Tsai MF, Thorat RS, et al. Endothelial Function and Postprandial Glucose Control in Response to Test-Meals Containing Herbs and Spices in Adults With Overweight/Obesity. Front Nutr. 2022;9:811433. https://doi.org/10.3389/fnut.2022.811433
- Nakayama H, Tsuge N, Sawada H, et al. A single consumption of curry improved postprandial endothelial function in healthy male subjects: a randomized, controlled crossover trial. Nutr J. 2014;13:67. https://doi.org/10.1186/1475-2891-13-67
- Raghu K, Surya RJ, Rani PK, et al. Incidence and Progression of Diabetic Retinopathy in Urban India: Sankara Nethralaya Diabetic Retinopathy Epidemiology and Molecular Genetics Study, 15yr Follow up. Ophthalmic Epidemiol. 2024;1-9. https://doi.org/10.1080/09286586.2024.2419015
- Shang C, Lin H, Fang X, et al. Beneficial effects of cinnamon and its extracts in the management of cardiovascular diseases and diabetes. Food Funct. 2021;12(24):12194-12220. https://doi.org/10.1039/d1fo01935j
- Azimi P, Ghiasvand R, Feizi A, et al. Effect of cinnamon, cardamom, saffron and ginger consumption on blood pressure and a marker of endothelial function in patients with type 2 diabetes mellitus: A randomized controlled clinical trial. Blood Press. 2016;25(3):133-140. https://doi.org/10.3109/08037051.2015.1111020
- Oak MH, Auger C, Belcastro E, et al. Potential mechanisms underlying cardiovascular protection by polyphenols: Role of the endothelium. Free Radic Biol Med. 2018;122:161-170. https://doi.org/10.1016/j.freeradbiomed.2018.03.018
- Yamagata K, Tagami M, Yamori Y. Dietary polyphenols regulate endothelial function and prevent cardiovascular disease. Nutrition. 2015;31(1):28-37. https://doi.org/10.1016/j.nut.2014.06.010
- Medina-Remón A, Casas R, Tresserra-Rimbau A, et al. Polyphenol intake from a Mediterranean diet decreases inflammatory biomarkers related to atherosclerosis: a substudy of the PREDIMED trial. Br J Clin Pharmacol. 2017;83(1):114-128. https://doi.org/10.1111/bcp.12986
- Amiot MJ, Riva C, Vinet A. Effects of dietary polyphenols on metabolic syndrome features in humans: a systematic review. Obes Rev. 2016;17(7):573-586. https://doi.org/10.1111/obr.12409
- Munir KM, Chandrasekaran S, Gao F, Quon MJ. Mechanisms for food polyphenols to ameliorate insulin resistance and endothelial dysfunction: therapeutic implications for diabetes and its cardiovascular complications. Am J Physiol Endocrinol Metab. 2013;305(6):E679-E686. https://doi.org/10.1152/ajpendo.00284.2013
- Hügel HM, Jackson N, May B, et al. Polyphenol protection and treatment of hypertension. Phytomedicine. 2016;23(2):220-231. https://doi.org/10.1016/j.phymed.2015.12.012
- Rodriguez-Mateos A, Le Sayec M, Cheok A. Dietary (poly)phenols and cardiometabolic health: from antioxidants to modulators of the gut microbiota. Proc Nutr Soc. 2024;1-11. https://doi.org/10.1017/S0029665124000012
- Ghosh SS, He H, Wang J, et al. Curcumin-mediated regulation of intestinal barrier function: The mechanism underlying its beneficial effects. Tissue Barriers. 2018;6(1):e1425085. https://doi.org/10.1080/21688370.2017.1425085
- Dey P. Gut microbiota in phytopharmacology: A comprehensive overview of concepts, reciprocal interactions, biotransformations and mode of actions. Pharmacol Res. 2019;147:104367. https://doi.org/10.1016/j.phrs.2019.104367
- Valdés L, Cuervo A, Salazar N, et al. The relationship between phenolic compounds from diet and microbiota: Impact on human health. Food Funct. 2015;6(8):2424–2439. https://doi.org/10.1039/c5fo00322aPubMed
- Clavel T, Henderson G, Engst W, et al. Phylogeny of human intestinal bacteria that activate the dietary lignan secoisolariciresinol diglucoside. FEMS Microbiol Ecol. 2006;55(3):471–478. https://doi.org/10.1111/j.1574-6941.2005.00057.xPubMed+1Frontiers+1
- García-Villalba R, Beltrán D, Espín JC, et al. Time course production of urolithins from ellagic acid by human gut microbiota. J Agric Food Chem. 2013;61(36):8797–8806. https://doi.org/10.1021/jf402498b
- Wang M, Yu F, Zhang Y, Chang W, Zhou M. The Effects and Mechanisms of Flavonoids on Cancer Prevention and Therapy: Focus on Gut Microbiota. Int J Biol Sci. 2022;18(4):1451-1475. https://doi.org/10.7150/ijbs.70431
- Koppel N, Maini Rekdal V, Balskus EP. Chemical transformation of xenobiotics by the human gut microbiota. Science. 2017;356(6344):eaag2770. https://doi.org/10.1126/science.aag2770
- Atkinson C, Frankenfeld CL, Lampe JW. Gut bacterial metabolism of the soy isoflavone daidzein: Exploring the relevance to human health. Exp Biol Med (Maywood). 2005;230(3):155–170. https://doi.org/10.1177/153537020523000302
- Jabczyk M, Nowak J, Hudzik B, Zubelewicz-Szkodzińska B. Curcumin and Its Potential Impact on Microbiota. Nutrients. 2021;13(6):2004. https://doi.org/10.3390/nu13062004PubMed
- Zhou M, Li R, Hua H, et al. The role of tetrahydrocurcumin in disease prevention and treatment. Food Funct. 2024;15(13):6798-6824. https://doi.org/10.1039/D3FO05739A
- Luo M, Wong S, Thanuphol P, et al. Isolation and Identification of Human Gut Bacteria Capable of Converting Curcumin to Its Hydrogenated Metabolites. J Agric Food Chem. 2024;72(37):20410-20418. https://doi.org/10.1021/acs.jafc.4c03828
- Burapan S, Kim M, Han J. Curcuminoid Demethylation as an Alternative Metabolism by Human Intestinal Microbiota. J Agric Food Chem. 2017;65(16):3305-3310. https://doi.org/10.1021/acs.jafc.7b01152
- Živanović N, Lesjak M, Simin N, Srai SKS. Beyond Mortality: Exploring the Influence of Plant Phenolics on Modulating Ferroptosis—A Systematic Review. Antioxidants (Basel). 2024;13(3):334. https://doi.org/10.3390/antiox13030334
- Liu J, Deng L, Qu L, et al. Herbal medicines provide regulation against iron overload in cardiovascular diseases: Informing future applications. J Ethnopharmacol. 2024;326:117941. https://doi.org/10.1016/j.jep.2024.117941
- Wang T, Liu M, Li X, et al. Naturally-derived modulators of the Nrf2 pathway and their roles in the intervention of diseases. Free Radic Biol Med. 2024;225:560-580. https://doi.org/10.1016/j.freeradbiomed.2024.09.035
- Wadowski P, Juszczak M, Woźniak K. NRF2 Modulators of Plant Origin and Their Ability to Overcome Multidrug Resistance in Cancers. Int J Mol Sci. 2024;25(21):11500. https://doi.org/10.3390/ijms252111500
- Andrés CMC, Pérez de la Lastra JM, Bustamante Munguira E, et al. Electrophilic Compounds in the Human Diet and Their Role in the Induction of the Transcription Factor NRF2. Int J Mol Sci. 2024;25(6):3521. https://doi.org/10.3390/ijms25063521
- Cortez N, Villegas C, Burgos V, et al. Adjuvant Properties of Caffeic Acid in Cancer Treatment. Int J Mol Sci. 2024;25(14):7631. https://doi.org/10.3390/ijms25147631
- Zhang M, Liu J, Yu Y, et al. Recent Advances in the Inhibition of Membrane Lipid Peroxidation by Food-Borne Plant Polyphenols via the Nrf2/GPx4 Pathway. J Agric Food Chem. 2024;72(22):12340-12355. https://doi.org/10.1021/acs.jafc.4c00523
- Cascajosa-Lira A, Prieto AI, Pichardo S, et al. Protective effects of sulforaphane against toxic substances and contaminants: A systematic review. Phytomedicine. 2024;130:155731. https://doi.org/10.1016/j.phymed.2023.155731
- Ochar K, Iwar K, Nair VD, et al. The Potential of Glucosinolates and Their Hydrolysis Products as Inhibitors of Cytokine Storms. Molecules. 2024;29(20):4826. https://doi.org/10.3390/molecules29204826
- Raeis-Abdollahi E, Raise-Abdullahi P, Rashidy-Pour A, et al. Coffee’s protective mechanisms against neurodegeneration. Prog Brain Res. 2024;288:167-200. https://doi.org/10.1016/bs.pbr.2024.06.017
- Nguyen V, Taine EG, Meng D, et al. Chlorogenic Acid: A Systematic Review on the Biological Functions, Mechanistic Actions, and Therapeutic Potentials. Nutrients. 2024;16(7):924. https://doi.org/10.3390/nu16070924
- Datta S, Ghosh S, Bishayee A, Sinha D. Flexion of Nrf2 by tea phytochemicals: A review on the chemopreventive and chemotherapeutic implications. Pharmacol Res. 2022;182:106319. https://doi.org/10.1016/j.phrs.2022.106319
- Joshi P, Bisht A, Paliwal A, et al. Recent updates on clinical developments of curcumin and its derivatives. Phytother Res. 2023;37(11):5109-5158. https://doi.org/10.1002/ptr.7780
- Satoh T, Trudler D, Oh CK, Lipton SA. Potential Therapeutic Use of the Rosemary Diterpene Carnosic Acid for Alzheimer’s Disease, Parkinson’s Disease, and Long-COVID through NRF2 Activation to Counteract the NLRP3 Inflammasome. Antioxidants (Basel). 2022;11(1):124. https://doi.org/10.3390/antiox11010124
- Colín-González AL, Santana RA, Silva-Islas CA, et al. The antioxidant mechanisms underlying the aged garlic extract- and S-allylcysteine-induced protection. Oxid Med Cell Longev. 2012;2012:907162. https://doi.org/10.1155/2012/907162
- Li Y, Zhu X, Wang K, et al. The potential of Ginkgo biloba in the treatment of human diseases and the relationship to Nrf2-mediated antioxidant protection. J Pharm Pharmacol. 2022;74(12):1689-1699. https://doi.org/10.1093/jpp/rgac072
- Borhani-Haghighi A, Mallahzadeh A. A comprehensive review on the neuroprotective potential of resveratrol in ischemic stroke. Heliyon. 2024;10(14):e34121. https://doi.org/10.1016/j.heliyon.2024.e34121
- Belka M, Gostyńska-Stawna A, Stawny M, Krajka-Kuźniak V. Activation of Nrf2 and FXR via Natural Compounds in Liver Inflammatory Disease. Int J Mol Sci. 2024;25(20):11213. https://doi.org/10.3390/ijms252011213
- She J, Gu T, Pang X, et al. Natural Products Targeting Liver X Receptors or Farnesoid X Receptor. Front Pharmacol. 2022;12:772435. https://doi.org/10.3389/fphar.2021.772435
- Liu Y, Huang K, Zhang Y, et al. Dietary polyphenols maintain homeostasis via regulating bile acid metabolism: a review of possible mechanisms. Food Funct. 2023;14(21):9486-9505. https://doi.org/10.1039/D3FO02471G
- Barbalho SM, Leme Boaro B, da Silva Camarinha Oliveira J, et al. Molecular Mechanisms Underlying Neuroinflammation Intervention with Medicinal Plants: A Critical and Narrative Review of the Current Literature. Pharmaceuticals (Basel). 2025;18(1):133. https://doi.org/10.3390/ph18010133
- Navarro E, Esteras N. Multitarget Effects of Nrf2 Signalling in the Brain: Common and Specific Functions in Different Cell Types. Antioxidants (Basel). 2024;13(12):1502. https://doi.org/10.3390/antiox13121502
- Cazalla E, Cuadrado A, García-Yagüe ÁJ. Role of the transcription factor NRF2 in maintaining the integrity of the Blood-Brain Barrier. Fluids Barriers CNS. 2024;21(1):93. https://doi.org/10.1186/s12987-024-00578-1