Healthy soil is alive, teaming with bacteria, fungi, insects and all sorts of life. This insightful article gives a deep dive on soil health, why it matters for human health, and how to restore our lands.
What is soil health?
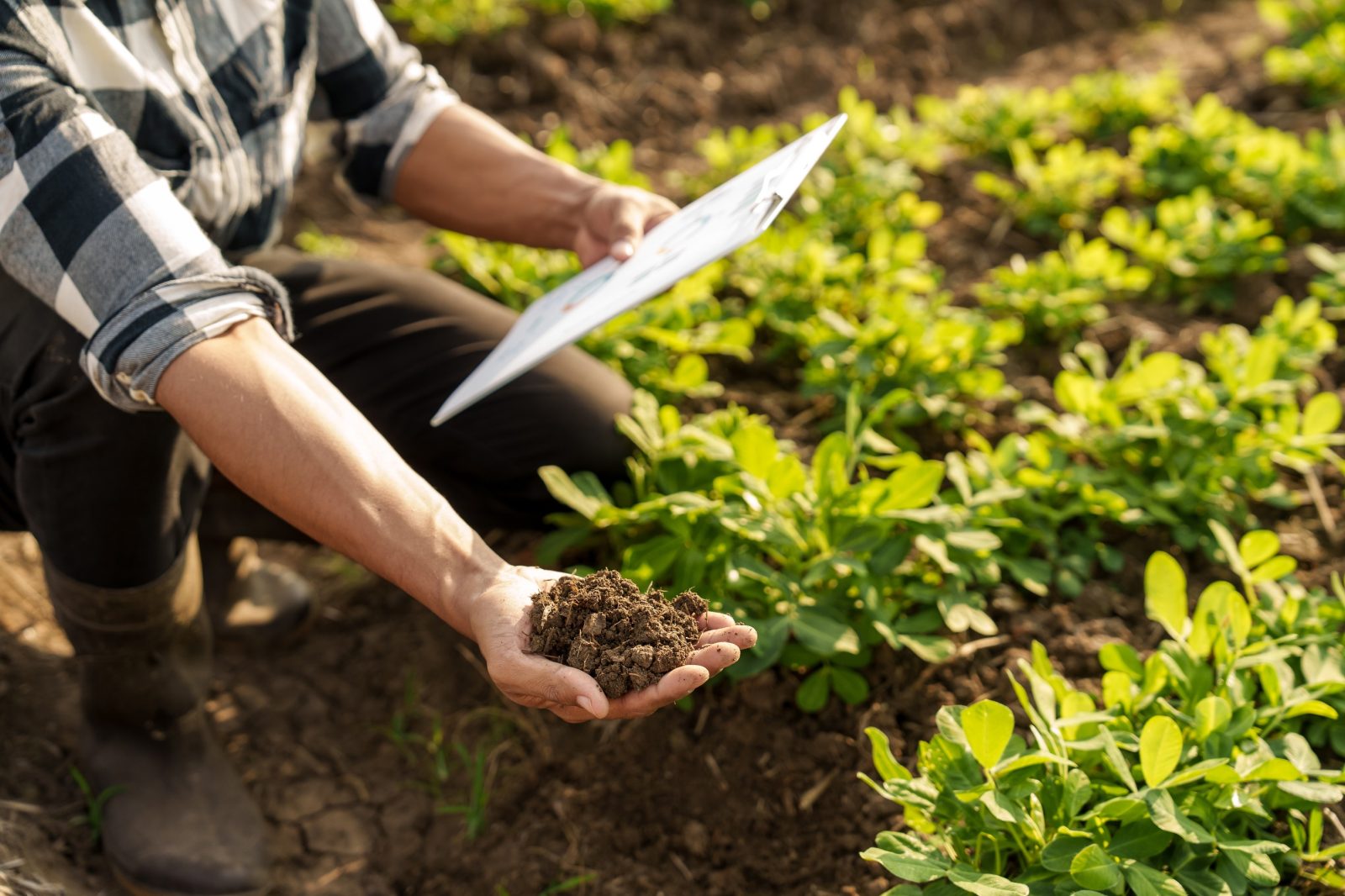
This is a much debated topic but the general consensus is that it is ‘the capacity of soil to function as a living system, within ecosystem and land-use boundaries, to sustain plant and animal productivity, maintain or enhance water and air quality, and support human health and habitation.’ (4)
If you dig a little deeper soil health is largely measured by the nutrients it contains, its texture, its capacity to drain, and a whole host of other physical and chemical properties. More often than not, in modern agricultural and horticultural settings, and especially at gardening and allotment scale, the biological side of soil health is just a footnote, if not ignored completely. And yet it is the biological story that links most intimately and intrinsically to the gut microbiome and human health.
What is the biological story?
Where to start? It would seem logical to discuss biology in the context of plants given that ultimately our health depends on what we consume directly, or indirectly via consumption of animal products. But that misses the overwhelmingly vital and fundamental contribution of the Earth’s microorganisms. Indeed, plants owe their evolution and crucially their healthy functioning, to microorganisms. After all, the archaea, bacteria, fungi and protists were around for some 2 billion years before plants even existed (5). These tiny engineers and biogeochemists evolved, diversified, competed and cooperated to create practically every metabolic substance, interaction, and pathway underpinning all later forms of life.
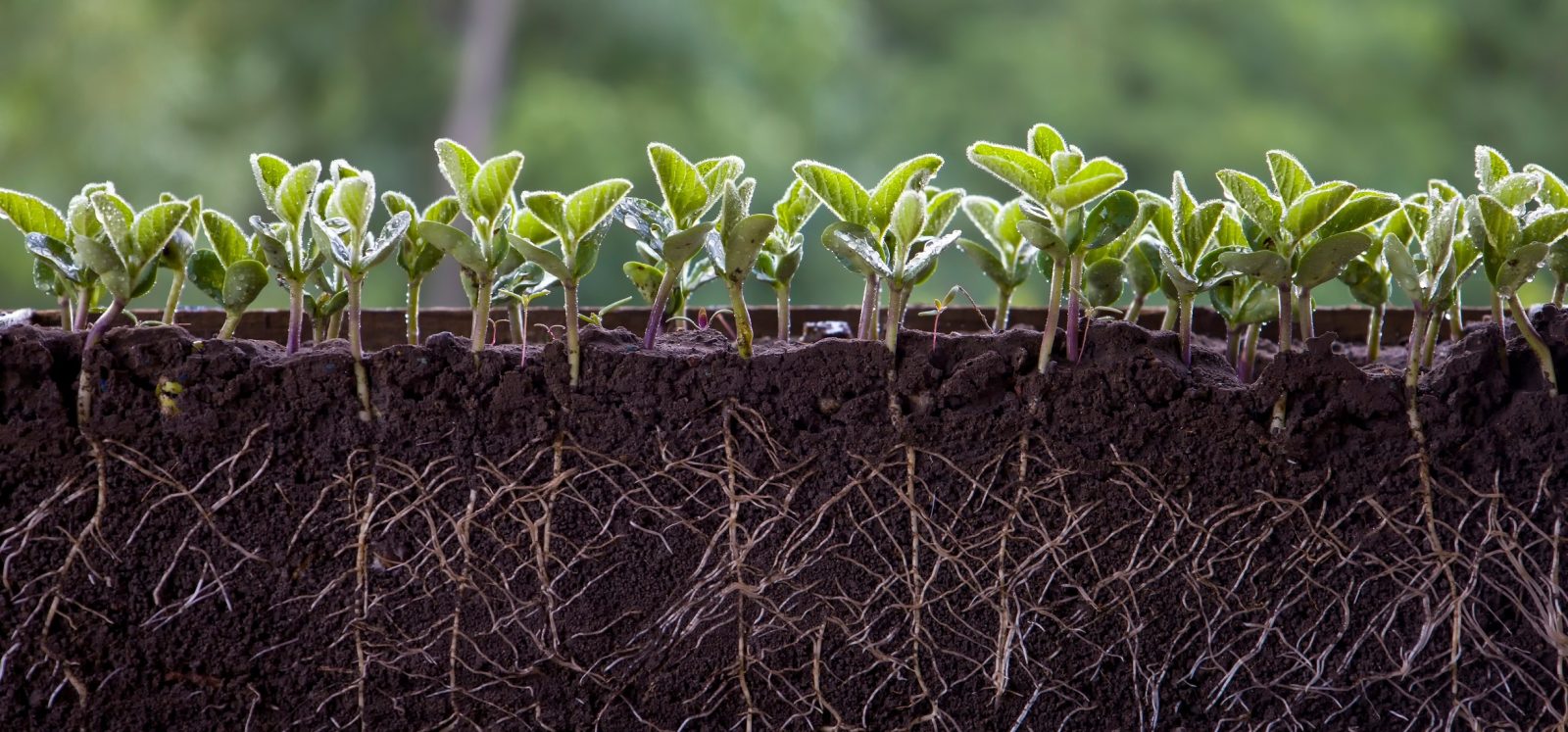
A metabolic pathway is a series of biochemical processes in cells that lead to a certain product or change in the cell, including the manufacture and use of enzymes and even turning genes on and off. For example, around 3.5 billion years ago bacteria invented photosynthesis as a means of capturing energy from the sun to synthesise food from carbon dioxide and water (6). The by-product of this process was oxygen, which gradually oxygenated the oceans and later the atmosphere.
In the late 1960s an American evolutionary biologist, Lynn Margulis, was researching the origins of eukaryotes, cells that contain a nucleus and various ‘organelles’ with specific functions such as the energy-producing mitochondria that are present in every cell of animals and plants (blood ‘cells’ excluded) (7). Margulis proposed that the organelles were once free-living, single-celled prokaryotic bacteria that had been consumed by larger, predatory bacteria. Doubtless, most mitochondria-type bacteria would have met their untimely death in this manner but some, it is thought, had the capacity to resist digestion and over time became an integral contributor to the cell. Importantly, the larger cell could rely on its partner cell for energy production and divert resources to other growth objectives.
Margulis’s theory gained widespread support when in later decades researchers discovered that mitochondria possessed DNA that proved their bacterial origins. The same is the case for other organelles and also bacterial plastids that today form chloroplasts in plants. And so it would follow that humans also owe their existence and health to microorganisms.
How does the soil food web work?
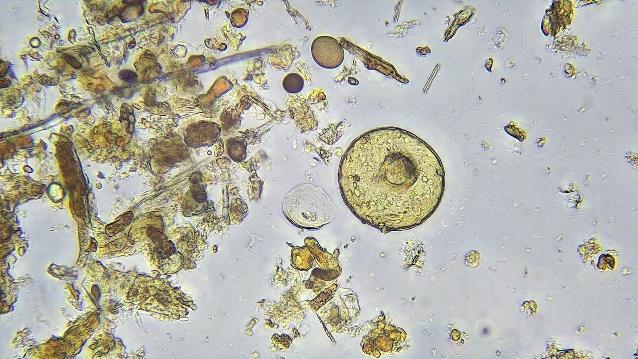
Plants divert up to 30% of their hard-won photosynthetic metabolites to their roots where they seep as ‘exudates’ into the rhizosphere, the thin zone a few millimetres thick surrounding the roots (8). These exudates attract ‘designer’ consortia of bacteria and fungi specific to each plant type. The intense bacterial activity in the rhizosphere in turn attracts predatory protozoans and nematodes. In the ensuing feast, digestion and excretion, excess nutrients such as nitrogen and phosphorous and many vital trace elements are released in bioavailable form into the soil solution and these are taken up by the plants (1). In addition, certain fungi referred to as mycorrhizal fungi (myco = fungus, rhizae = root) make physical connections with plant roots and shuttle mineral nutrients and water to the plant in exchange for carbon sugar. Certain bacteria called rhizobia also make physical connection with the roots of legumes and form nodules in which they fix nitrogen into plant-available form.
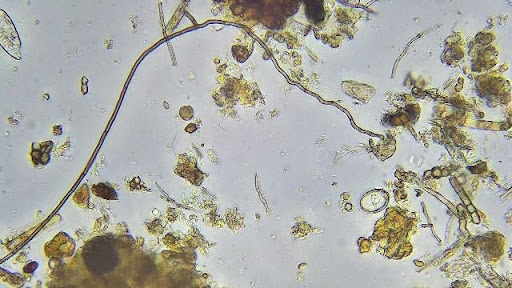
Researchers have known about mycorrhizae since 1809 (9), and rhizobia since the 1880’s, and from the 1950’s onwards strains that can be economically grown in sufficient concentrations have formed an integral part of the fertiliser routine of many farms. But in the last decade researchers have also discovered that plants actively draw bacteria into their roots where they ‘hit’ them with secretions that break down their cell walls but without killing them. The rich nutrients from the cell walls are absorbed by the plant cells. The ‘naked’ bacteria in response secrete the plant growth-promoting hormone ethylene which causes root hairs to grow and through these tiny tunnels they escape back into the fertile soil solution where they reform their cell walls. Many return to relive the experience in what has become known as the ‘rhizophagy cycle’ (rhizo = root, phagy = eat) (10). Dr James White suggests the majority of a plant’s nutrition is obtained in this way.
Continuing research has since discovered that bacteria are being partitioned into every type of plant cell where it appears they play a symbiotic role in support of plant health including, amongst others, nutrition, immune functioning, and signalling.
Quite clearly then, soil microorganisms are fundamental to plant health. If a plant is to realise full robust health it follows it must be supported by a fully functioning soil food web and by extension a fully functioning foliage food web.
What happens when the food web isn’t working?
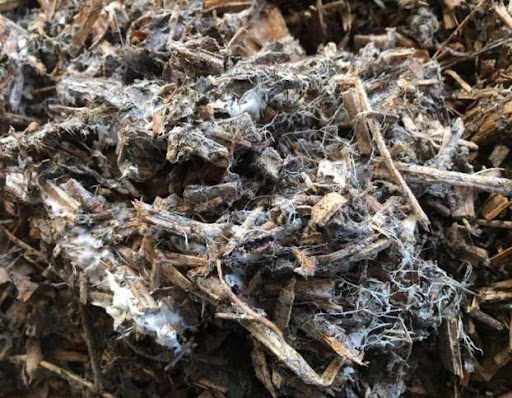
In soil degraded by ploughing or digging, or through the application of synthetic fertilisers and biocides, it is obvious that the major component of plant nutrition, evolved and perfected over 450 million years, is practically destroyed (11). Only bacteria survive, and then in depleted numbers and types. In these soils plants cannot attain robust health. They will still grow, even large if there is an abundance of bacteria-derived nitrate, but size is not a sign of good health.
The plant will expend precious energy attempting to acquire mineral nutrients in bioavailable form. However, ultimately the plant will be depleted of vital trace elements and be prone to disease and insect attack. And if the plant is depleted in mineral nutrients and vitamins, then those who eat that plant will also experience the effects of malnutrition. You can read more about the effects of modern agriculture on nutrition in our article “Crap food leads to crap health: Why do we put up with it?”
How does this link to the gut microbiome?
The main microbial players are the same, just different strains and different ratios because the soil is largely an aerobic environment and our guts are essentially anaerobic. In terms of bacteria (which dominate, but that is not to downplay the role of gut fungi and protists) these players are the Bacillota (formerly the Firmicutes), the Bacteroidota, the Actinobacteria, and the Proteobacteria. The latter two groups are dominant in the soil and the former two are significantly subordinate; the reverse is true for the human gut. Nevertheless, all the metabolic support mechanisms of soil and gut are directly comparable. As stated above a healthy gut depends on a healthy gut microbiome. Just as it is with malnourished plants from a depleted soil microbiome, if we eat those malnourished plants our gut flora and fauna will be depleted in the essential elements that help them function to produce the metabolites that support a healthy human.
What are the implications for human health?
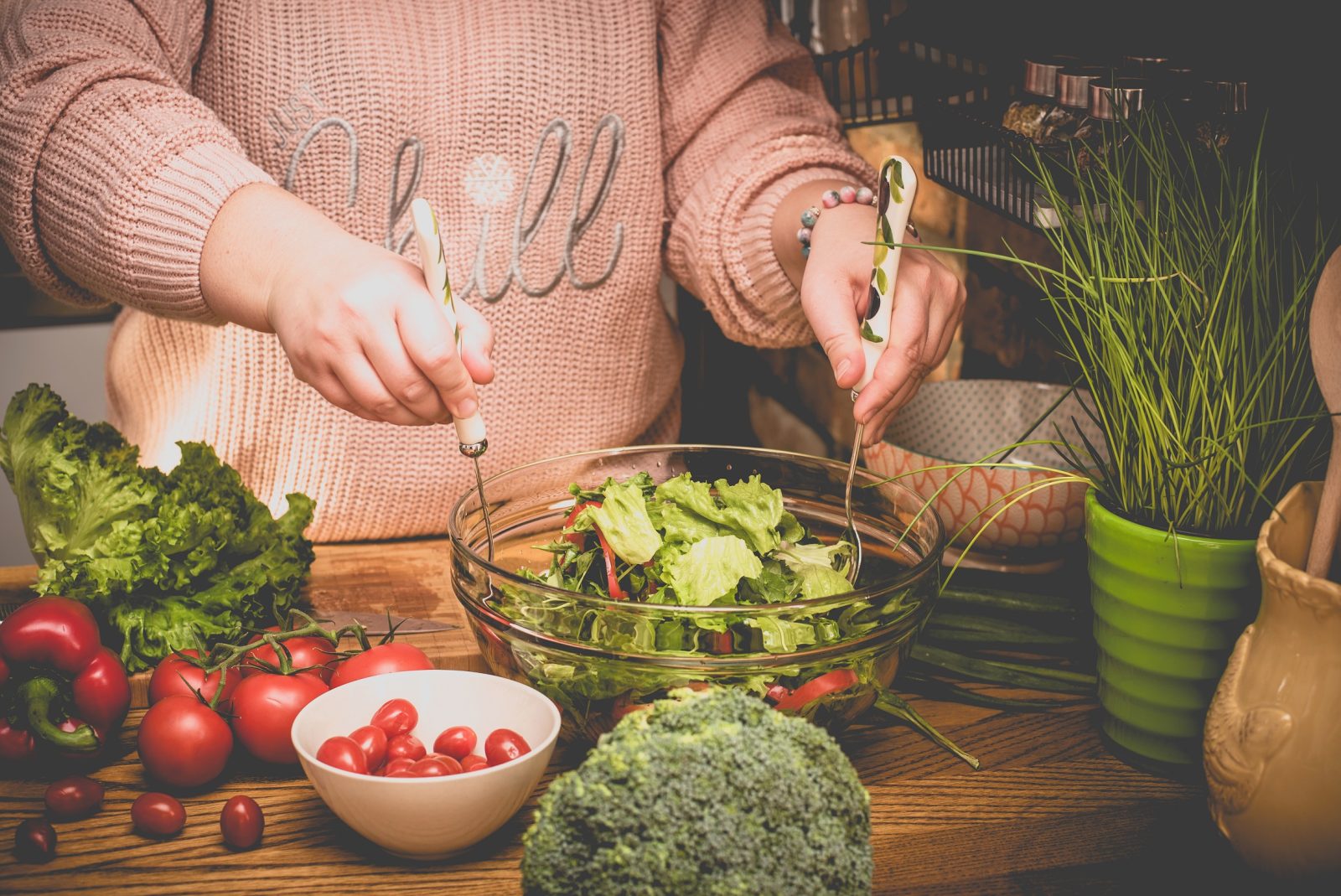
It is considered that this disconnect with nature, this catastrophic breakdown in the biological bridge between soil, plant and gut microbiomes, is in part responsible for the significant sharp rises in inflammatory ill-health and other maladies over the last few decades (ultra-processed foods, pesticide residues, and pollution doubtless being other causal factors. And overuse of antibiotics is the equivalent of reckless use of agricultural biocides). Looking at this from another perspective, if our food was the full ticket of essential nutrients and vitamins in natural bioavailable form we would be in far better health to cope with other stresses, biotic or abiotic that life throws at us. Our article “The impact of pesticides on the gut microbiome: A closer look at health risks” discusses this important issue.
And that goes equally for animals nurtured for human consumption. Farm animals are increasingly in need of mineral supplements, including vitamin B12, also called cobalamin due to the metal cobalt that is crucial to its formation. With the overuse of synthetic fertilizers and biocides it is considered that the microorganisms of the soil food web that support the pastures have been severely depleted. Given that it is specific bacteria alone that manufacture vitamin B12 (12), and research indicating that fungi help shuttle bacteria to and from the mineral ‘coal-face’, the depletion of both microorganisms in the soil has only one outcome for the gut.
Anything else?
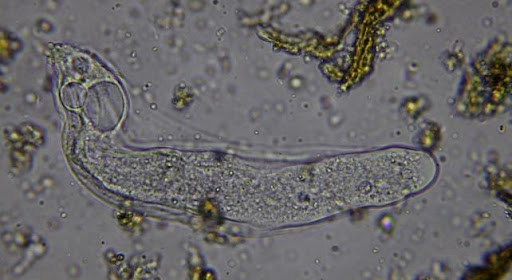
Let us not forget the disruption to the seed microbiome or the effects of selective breeding. A human mother passes on her healthy gut microbiome to her newborn through natural childbirth, or at least that is what should happen, the biological principle being if mum thrived in her environment with her consortia of gut bugs then so too will the baby. But if mum’s gut flora and fauna are depleted then the baby will be deprived of the optimal start in life and may never recover that ground.
Selective breeding to express certain economically desired traits such as bigger, brighter blooms, less bitter brassicas, or more milk production also usually come at a cost to the natural microbiome. For example researchers working on drought-tolerant wheat varieties noticed a greater susceptibility to fungal pathogens.
Scratching the surface
This account, whilst attempting to cover many bases, in reality just scratches the surface of the complexity of connectedness and interactions between the various microorganism functional groups and their habitat microbiomes, which furthermore can be considered in larger groups referred to as holobiomes. Whilst seemingly incomprehensible, what is without a shadow of a doubt is this intricate system developed over many millions of years, underpins the health of higher organisms, although I sometimes wonder just who are the ‘higher’ organisms.
Where to begin with caring for our land?

So there we are, looking out over our humble plot of land; it might be your farm, your allotment, or your garden, eyes opened to the possibility of doing something incredible. I’m not just talking about producing some of your own food and flowers. I’m talking about producing food of the highest nutrient density to secure your good health, and shrubs and flowers of such sensory brilliance that your general wellbeing is truly boosted. Even more, you could be doing your bit to drag some of that pesky atmospheric CO2 back into the ground where it can do some serious good for the rest of nature.
The last recorded statistics from 1998 show there were around 250,000 allotment plot-holders in the UK, and 23 million back/front gardens (2); that’s just over 500,000 hectares (a hectare is a plot of land 100m long by 100m wide). Applications for allotments after the coronavirus pandemic soared with some areas experiencing a 300% increase in applications for a plot (3). Add to that 5 million hectares of cropable arable land and you have a huge area with the potential to make a difference to health and climate.
The stock-take: Understanding your soil
The first thing to do is to gain an understanding of how well, or poorly, your soil food web is doing. Remember, it is via the soil food web that virtually every plant gains its vitality. So have we provided the right habits and conditions for our tiny engineers and biogeochemists to operate at peak performance? Have we been nurturing them with the right foodstuffs i.e. composts, extracts, and teas? Indeed are the microbes there in the first place in decent numbers and diversity?
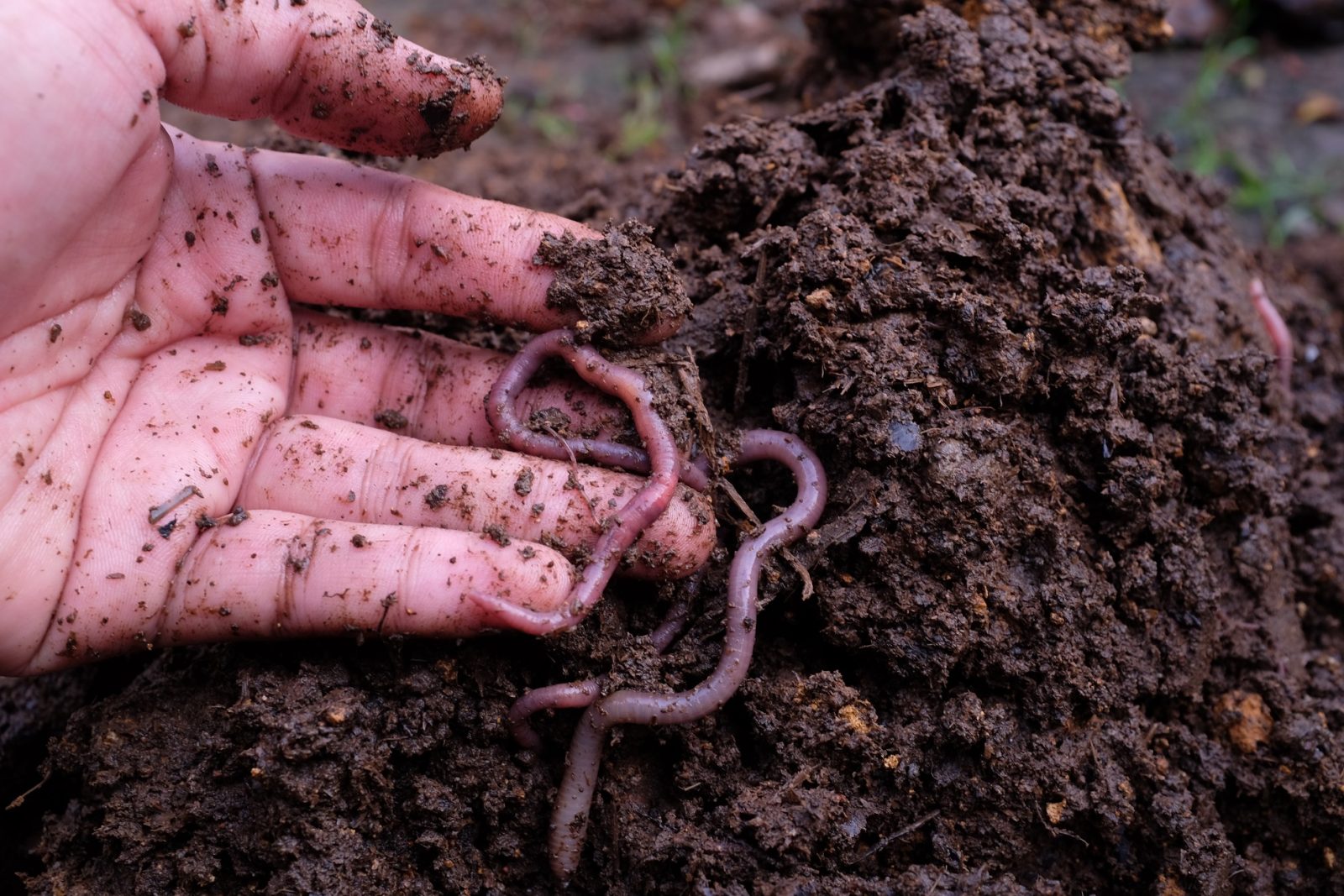
To understand the last point does not require a microscope, although if you can afford one it will little short of changing your life! Think of the Serengeti with its own complete food web. There are photosynthetic primary producers like trees, shrubs and grasses, and cyanobacteria and algae in lakes and rivers. Next along the food web you have the enormous numbers of primary consumers such as grazing zebra, wildebeest, gazelles, and waterbuck. These are followed (quite literally) by secondary consumers such as cheetahs and leopards. Eventually, you reach the apex predator, the lion. Now, if there is little primary production going on there will be little reason for primary consumers to be there. And if there are no, or very few, primary consumers there will be no, or very few secondary consumers, and so on. Make sense? Turning to your soil, take a spade and create a square cut the width of your spade and a spade deep. Lift it out and gently tease the soil apart.
How many worms can you count? A healthy soil will have at least fifteen to twenty worms in that volume. The worms can be imagined as the lions of your soil food web. They will be there if they have plenty of microarthropods, nematodes, protozoans, bacteria and of course fungi and decaying organic matter to feed on i.e. a full soil food web (I’m presuming that if the full complement of microbes is present and functioning well that water is in good supply). If your worms have gone AWOL (Absent Wormout Leave) you ought to consider the levels of decaying organic matter (food!) in your soil, and the microscopic critters missing in action (although usually there will be a depleted population of bacteria hanging on to what small reserves of carbon and nitrogen they can muster).
We do have to take into account the time of year and transient environmental conditions that might naturally affect numbers including winter cold, summer drought, spring waterlogging etc. But overall, we know that a full complement of microorganisms is the plant’s natural and preferred (evolved) means of obtaining nutrients and other life-support services. These are called secondary metabolites and include phytochemicals that help keep pathogens at bay, for example the usually abundant and reliable Pseudomonas bacteria contain species that secrete hydrogen cyanide that degrades fusarium fungi which causes many plants to wilt! Interestingly it is oftentimes these secondary metabolites that have medicinal effects in humans too.
First response
There are other signs of a biologically depleted soil; compaction, weeds, waterlogging due to texture.
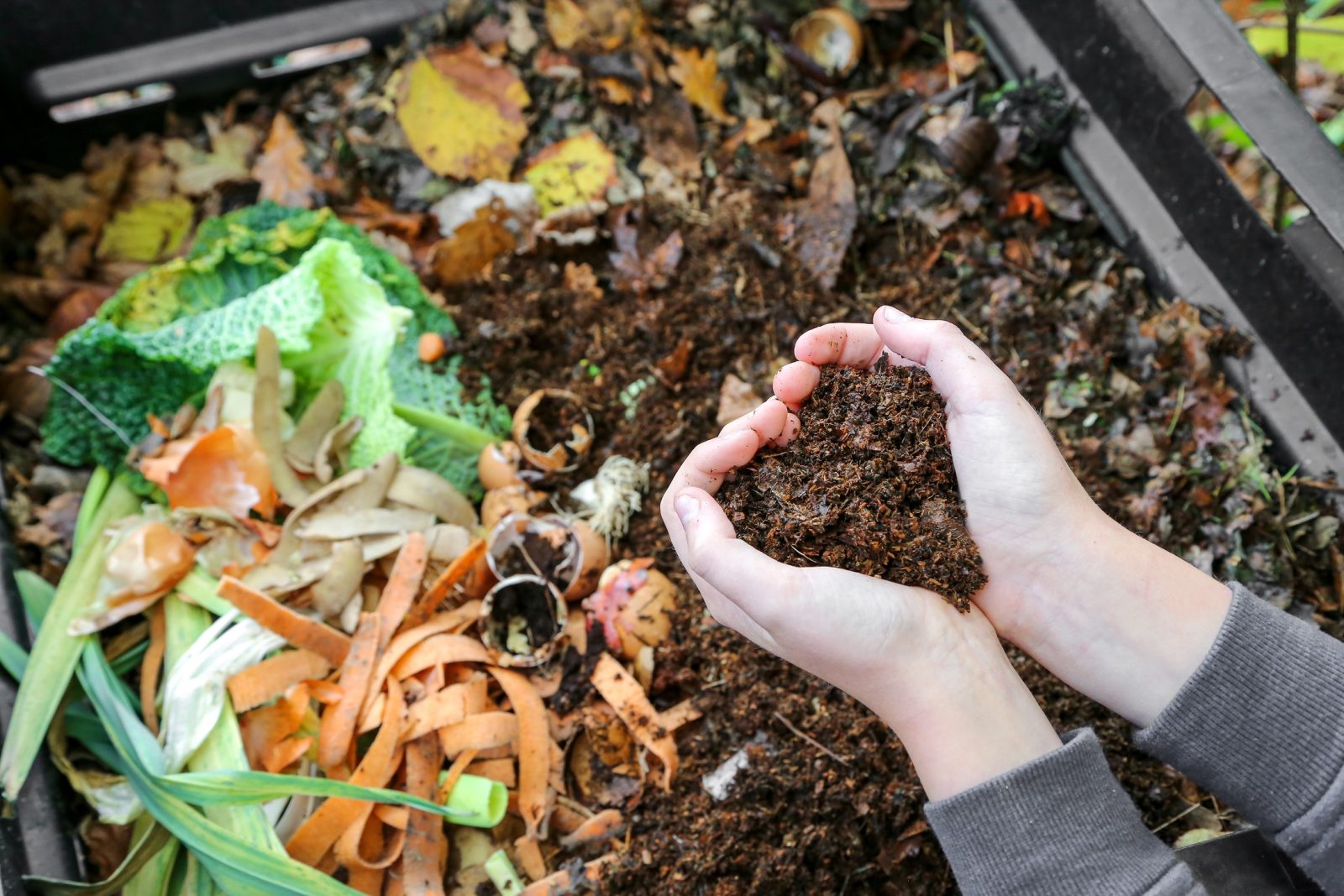
I should just explain what I mean by a biologically depleted soil because to some extent it depends on what you are trying to grow. It’s the old ‘square pegs in round holes’ dilemma. For most gardeners and allotmenteers the end-game is a general mix of vegetables, fruit, herbs, shrubs and/or flowers and this means a certain consortia of microorganisms that associate with those plants. But simply due to climate and geology it may be immensely difficult to achieve your goals without serious intervention. For example, if you want to grow potatoes on alkaline clay soils in the far west of the UK you would be better off building raised beds and filling them with organic-rich, sandy soil of low-ish pH and keeping your fingers crossed regards the temperature and rainfall. But those alkaline, wet, clay soils will be biologically brilliant for the plants nature has evolved via the fitted microorganisms to fill that niche, and for the animals that eat those plants.
Another key giveaway is the colour of your soil. A soil rich in decayed organic matter will be a dark chocolate brown or very dark grey colour. Anything pale (brown, orange, yellow, grey) will be lacking in essential foodstuffs for the primary consumers and this is where it all begins. This is the one time I would consider digging, to incorporate good quality, home-made compost. I would avoid municipal greenwaste and bagged so-called composts (really just mulches in my opinion which tend only to have bacteria within). It’s astonishing but really ought not to be surprising, the diversity of microbial life in a common or garden compost pile made of kitchen compost-caddy fillings, grass clippings, weeds, border prunings etc. As long as you have not added chemicals (including grass weed and feed) and have kept an eye on the browns versus greens (try and mix 50:50 for general applications), and have turned the pile once or twice for aeration, then I can assure you that after a year the bottom half metre or so of your compost pile will be a heaving soil food web metropolis.
You can read more about how to make your own compost in our article “how to make your own compost”.
Maintenance and supercharging your soil
Once established with good levels of organic matter it is simply a case of feeding with the homemade compost as a surface dressing every month to six weeks and ensuring it stays moist in extended dry weather. Recall you are creating the right habitat with basic food supply to get the soil food web nutrient cycling going, and periodically drafting in more of the soil microbes best fitted to your plants.
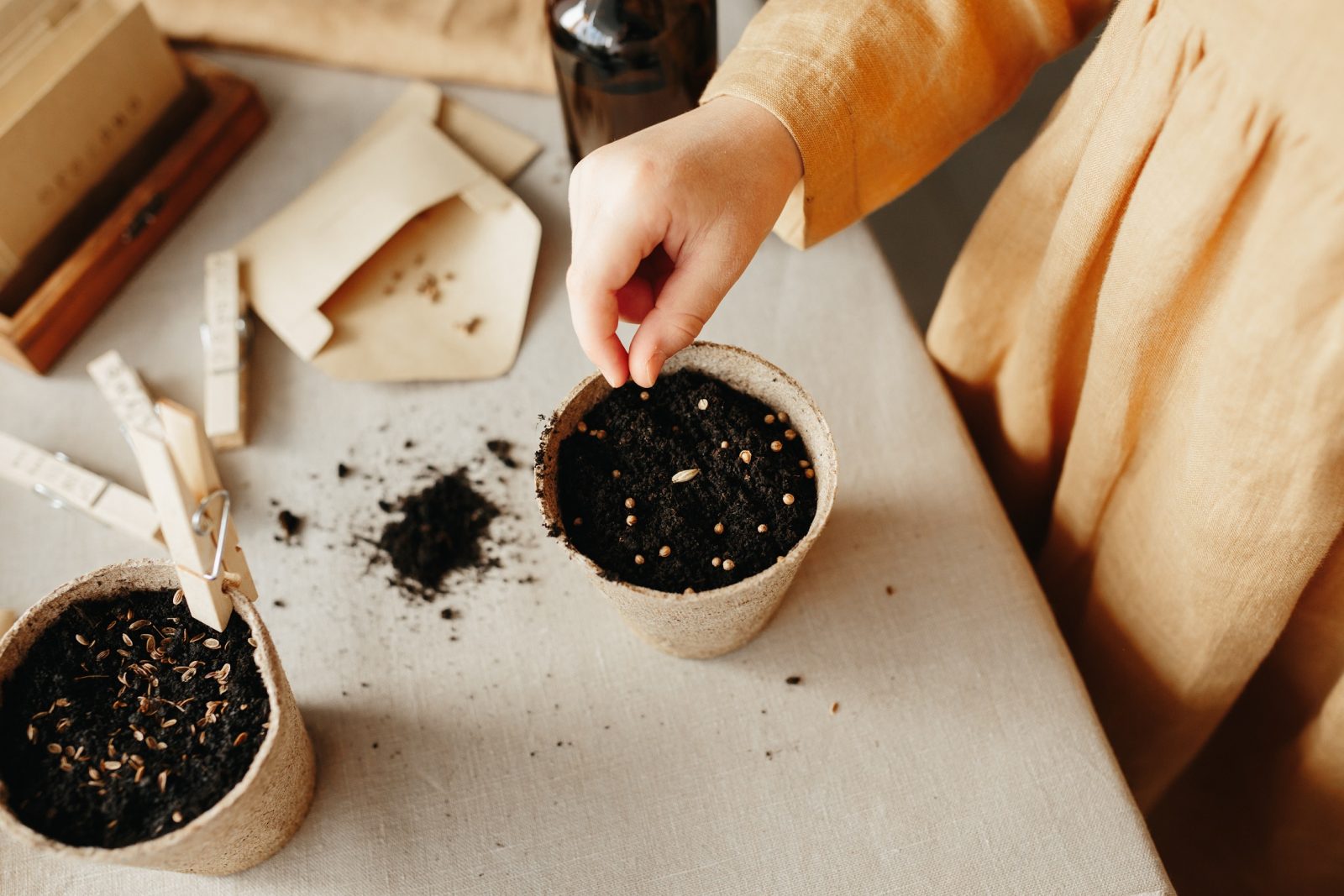
It is also worthwhile taking a few big handfuls of your good quality compost and soaking it in a bucket of tepid rainwater. Give it a good swish-around with a stick to knock the microbes off their perches, then strain the biologically rich, dark brown solution through a 400-micron mesh. You have just produced a wonderful, balanced fertilizer plus microbes, that can be used undiluted as a root-drench, or even used through a sprayer as a foliar feed and protectant (the microbes will cover the leaves helping to ensure pathogens cannot establish a foothold through the antivirals, antibiotics and antifungals they secrete).
In the early days of your rehabilitation (yes, I’m as much referring to the grower as to their soil) you could add a capful of seaweed extract if you had any doubt as to the nutrients immediately available to your plants. For arable farmers on the other hand, the ability to build up the basic levels of organic matter to kick start the biology is hampered by the lack of compost on the scale needed in a country with the least amount of natural woodland and hedgerow in Europe. We seem obsessed too with selecting breeds that maximize yields and minimize vegetative growth, all to make harvesting easier. Just think about all that free carbon and nitrogen given up by reducing wheat and rapeseed oil, for examples, from 6ft and 8ft heritage strains respectively to the thigh-high crops of today.
Sit back and enjoy the rewards of THEIR endeavours

As the fertility of your soil builds up you will find the microrganisms doing all of the work for you. They will fine-tune the structure of your soil, aerating through the development of micro- and macro-aggregates, they will connect with your plants through an assortment of fungi and bacteria offering a mind-blowing array of secondary metabolites and water. And as the soil food web operates at full capacity the nutrients your plants require will be made bioavailable in the optimal ratios.
As you munch through your nutrient-packed, low CO2-footprint lunch, looking out over your flower border brimming with wildlife, casually noticing that your asthma or psoriasis has improved, that your energy levels are greater, and how mentally relaxed you are, take a moment to consider also how much carbon you have sequestered in the process and what this might mean for the generations that follow.
References
- Soil Foodweb School. Dr. Elaine’sTM Soil Food Web School – EN – Regenerative Agriculture courses. Soil Food Web School – EN. Published May 3, 2023. https://www.soilfoodweb.com/
- Demand for Allotments. publications.parliament.uk. Published June 24, 1998. https://publications.parliament.uk/pa/cm199798/cmselect/cmenvtra/560/56008.htm
- Smithers R. Interest in allotments soars in England during coronavirus pandemic. The Guardian. https://www.theguardian.com/lifeandstyle/2020/aug/10/interest-in-allotments-soars-in-england-during-coronavirus-pandemic. Published August 11, 2020.
- Thangarajan R, Bolan N, Tian G, Naidu R, Kunhikrishnan A. Role of organic amendment application on greenhouse gas emission from soil. Science of the Total Environment. 2013;465:72-96. doi:10.1016/j.scitotenv.2013.01.031
- Alegado RA, King N. Bacterial influences on animal origins. Cold Spring Harbor Perspectives in Biology. 2014;6(11):a016162. doi:10.1101/cshperspect.a016162
- Photosynthesis more ancient than thought, and most living things could do it. Imperial News. Published March 14, 2016. https://www.imperial.ac.uk/news/171358/photosynthesis-more-ancient-than-thought-most/#:~:text=A%20new%20study%20by%20an,than%203.5%20billion%20years%20ago.
- Wikipedia contributors. Lynn Margulis. Wikipedia. Published online July 19, 2023. https://en.wikipedia.org/wiki/Lynn_Margulis
- Canarini A, Kaiser C, Merchant A, Richter A, Wanek W. Root exudation of primary metabolites: Mechanisms and their roles in plant responses to environmental stimuli. Frontiers in Plant Science. 2019;10. doi:10.3389/fpls.2019.00157
- University of Hawaii. Evaluating mycrorrhizal inoculum levels in soil. Published August 1980. https://www.ctahr.hawaii.edu/bnf/Downloads/Dissertations/Dowdle%20Thesis.htm
- White JF, Kingsley KL, Verma SK, Kowalski KP. Rhizophagy Cycle: An Oxidative Process in Plants for Nutrient Extraction from Symbiotic Microbes. Microorganisms. 2018;6(3):95. doi:10.3390/microorganisms6030095
- Evolution of land plants changed composition of Earth’s crust, study finds. News. Published August 30, 2022. https://www.abdn.ac.uk/news/16300/
- Fang H, Kang J, Zhang D. Microbial production of vitamin B12: a review and future perspectives. Microbial Cell Factories. 2017;16(1). doi:10.1186/s12934-017-0631-y